mass
amount
molar mass
concentration
solution volume
gas volume
molar gas volume
Avogadro
constant, L
number of
entities, N
CALCULATING ON THE SIDE OF SUCCESS – Module R(2028): APPLICATIONS OF STOICHIOMETRY
R. SOME MORE ADVANCED APPLICATIONS OF STOICHIOMETRY
R1. MASS % YIELD
R1.1 PRACTICAL LIMITATIONS PLACED UPON SYNTHETIC METHODS
In the stoichiometry calculations tackled so far, it has been assumed that, for the most part, all of the reactants are transformed into products, i.e., the reactions are considered to go to completion. Such an assumption has been necessary in order to arrive at an answer. In practice, however, this is the exception rather than the rule.
As with any lab-bench synthetic procedure, the quantity of product obtained experimentally in many industrial and commercial chemical processes – the yield or reaction yield – is one of the important measures of a successful chemical reaction.
The stoichiometric quantity of the desired product in
a reaction based on a specified reactant is referred to
as the theoretical or stoichiometric maximum yield.
It can be calculated by comparing the experimental yield of product obtained with what would have been generated had all of the reactants been converted, with no loss or waste.
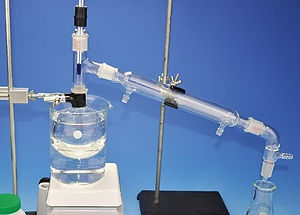
Obviously total conversion under ideal circumstances will be 100 %. In reality, however, this rarely happens.
Yields »100 % are termed quantitative, e.g., some inorganic precipitations come close to this ideal.
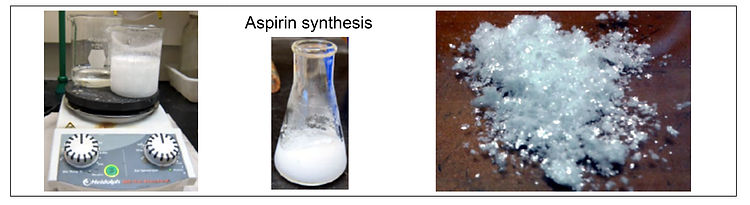
So, experimental yields obtained in chemical reactions are less than stoichiometric maximum yields
for a variety of reasons, some of the more obvious being as follows.
1.
Many reactions are incomplete and so the reactants are not converted quantitatively to products. Unless one or more products escape the reaction mixture, chemical reactions naturally reach an equilibrium position that is governed by the energy changes involved. If a reverse reaction occurs, both reactants and products will be found in an equilibrium mixture, e.g., when an alcohol esterifies with a carboxylic acids.

Two or more reactions may occur simultaneously, so that some reactant is converted via a side reaction to a different, undesired side product, e.g., combustion of hydrocarbons in
2.
oxides of nitrogen. And some of the fuel may vaporise, releasing unburnt hydrocarbons.
3.
Some of the product may be left behind in the process of separating it from excess reactants and therefore losses occur in the separation and purification of the desired product from the reaction mixture.
4.
The starting materials may not be pure, meaning that the quantities of reactants present are less than the masses weighed out. Impurities present in the starting material do not react to give desired product.

R1.2 DEFINITION OF % YIELD & KEY TERMINOLOGY

The stoichiometric maximum yield is the amount of a product predicted by a stoichiometric calculation based on the amounts of all reactants present, assuming that only one reaction occurs and that all of the limiting reactant is consumed, i.e., reacts completely.
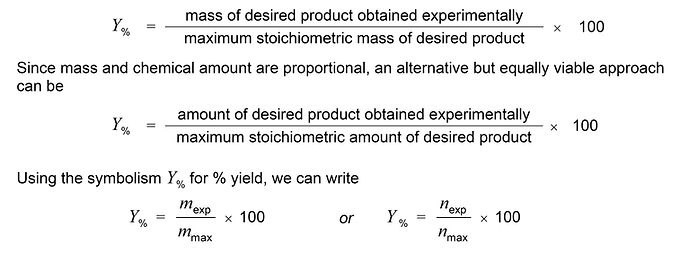
% yields are typically quoted to the nearest whole % given the likely variability inherent in the various practical procedures used to generate the experimental data.
R1.3 CALCULATION OF % YIELD: WORKED EXAMPLES
Often in such problems, the key step is calculating the maximum, i.e., stoichiometric, mass or chemical amount of the desired product – the denominator on the RHS of either of the above expressions. Most commonly, this is the quantity which a question does not provide.
The % yield of a chemical reaction is an important consideration in any commercial or industrial context – the higher a % yield, the more efficient the reaction.
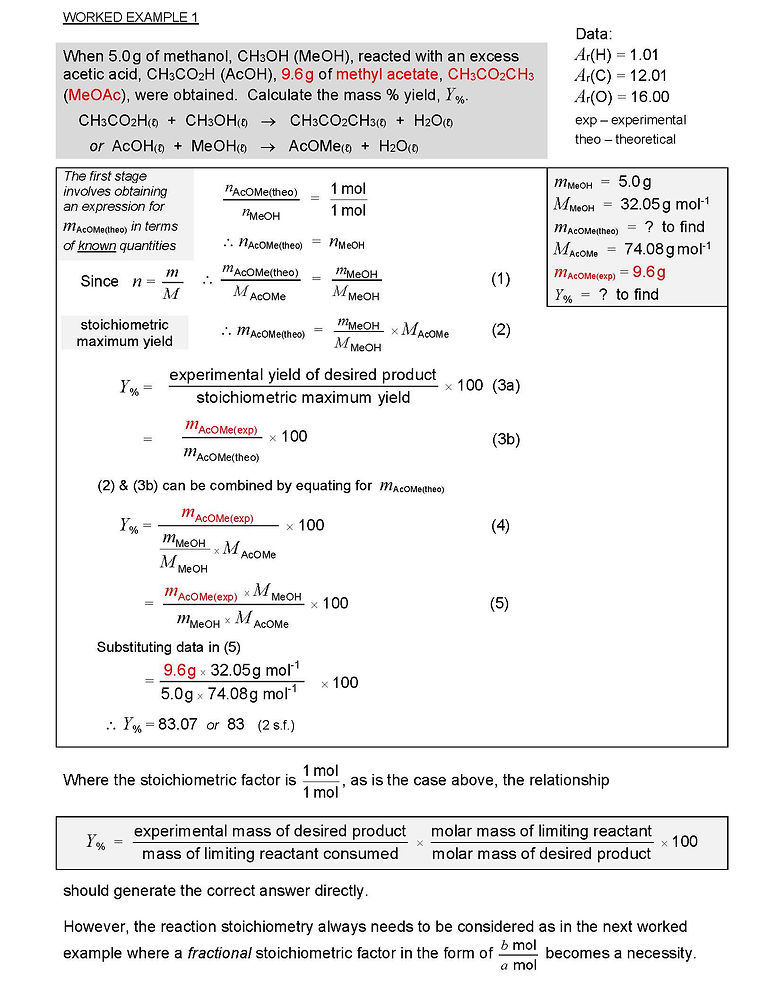

R1.4 CALCULATION OF % YIELD: PRACTICE PROBLEMS

